微通道板 (MCP)
微通道板 (MCP)
无法加载取货服务可用情况
MCP 的目标对象:电子、(+/-)离子、真空紫外线、X 射线
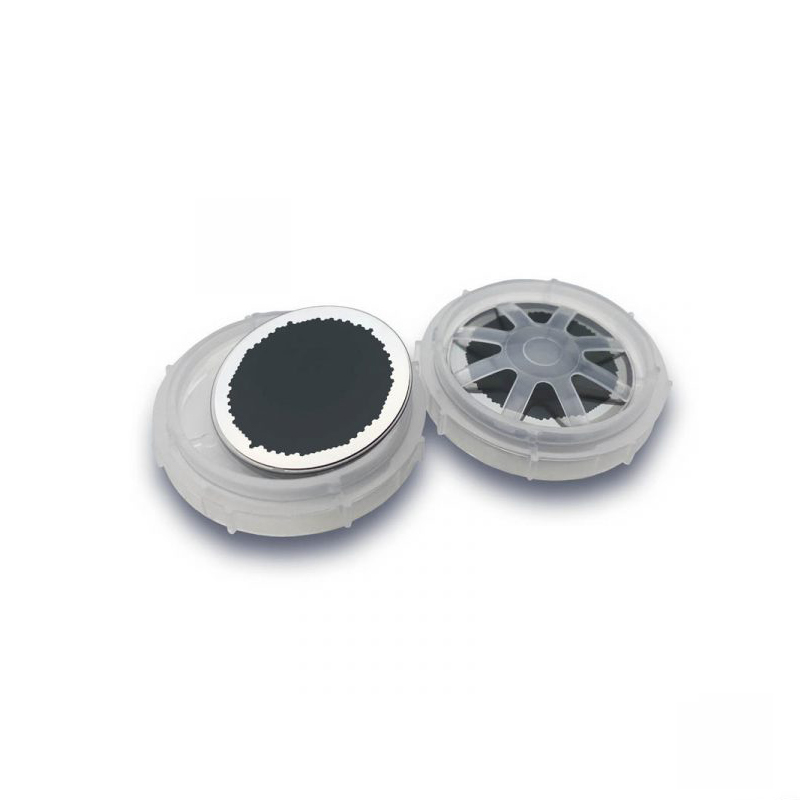
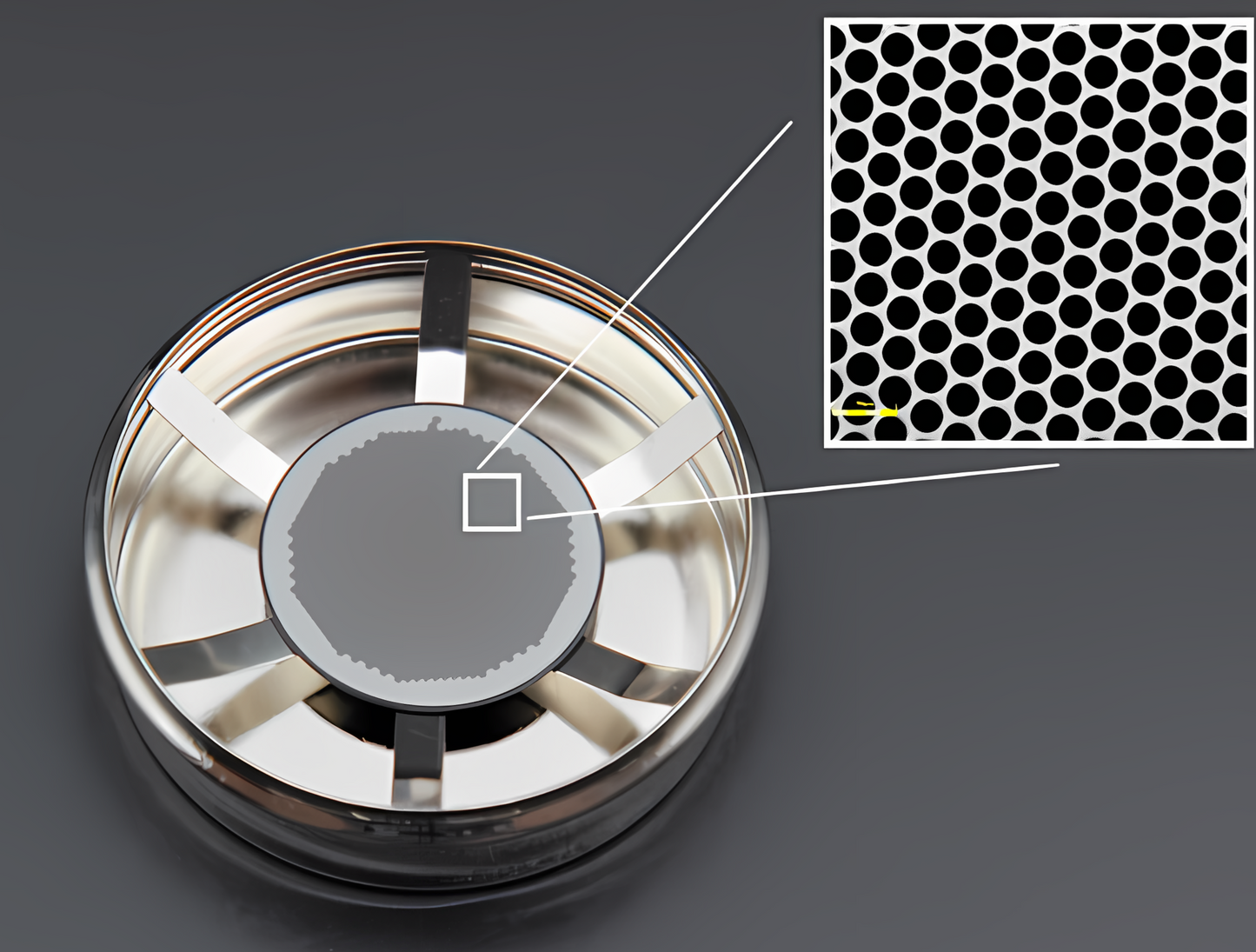
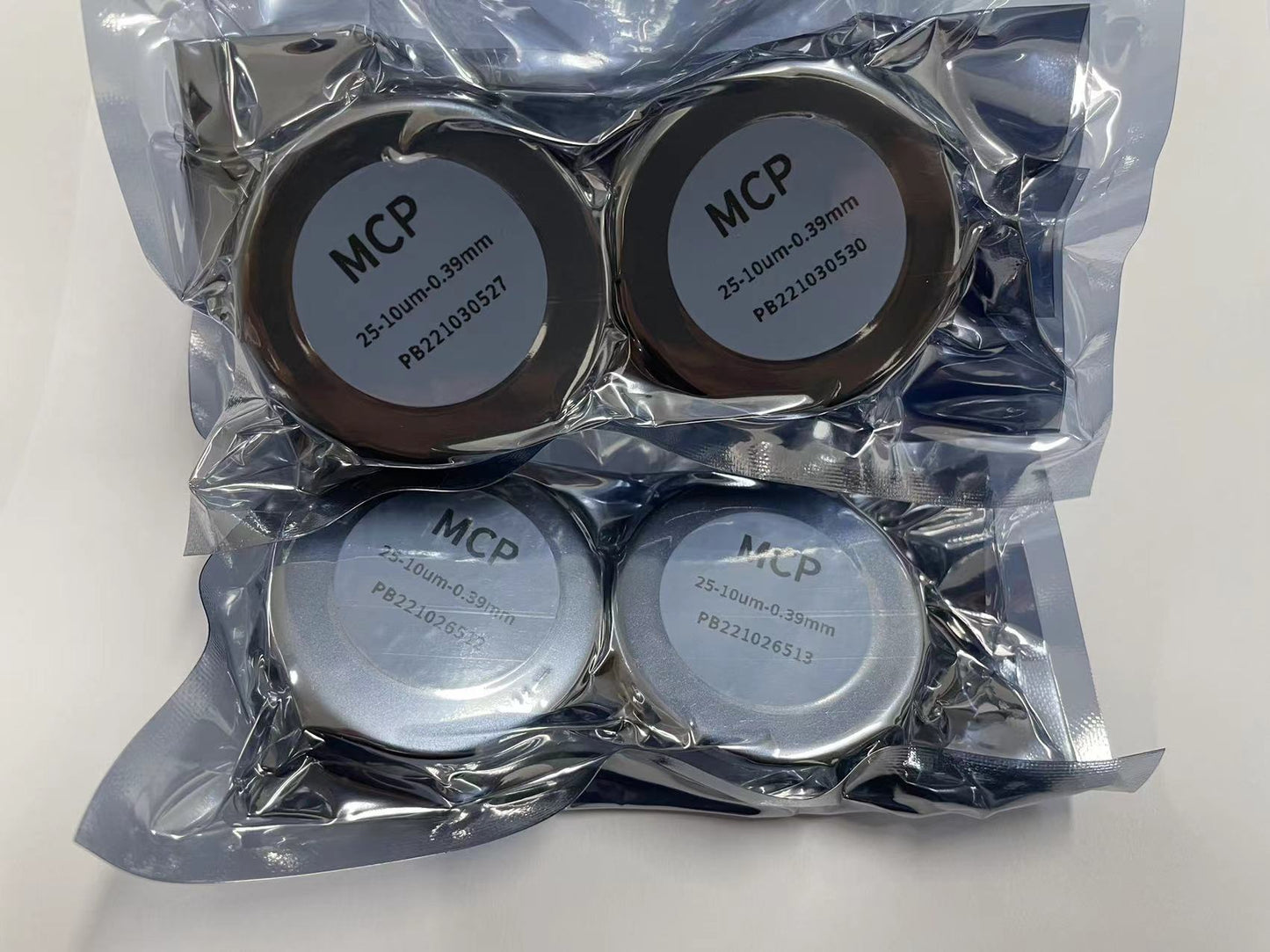
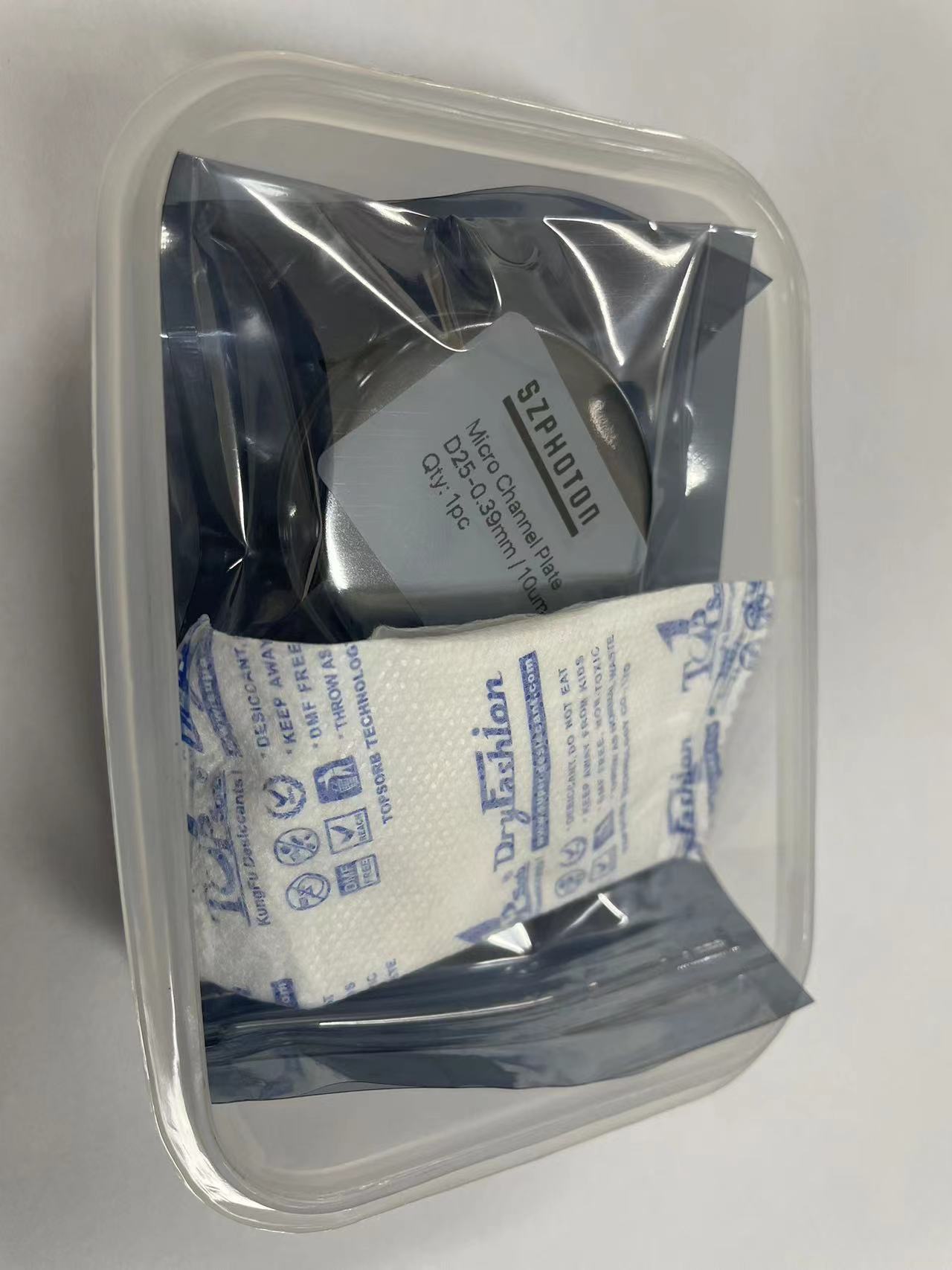
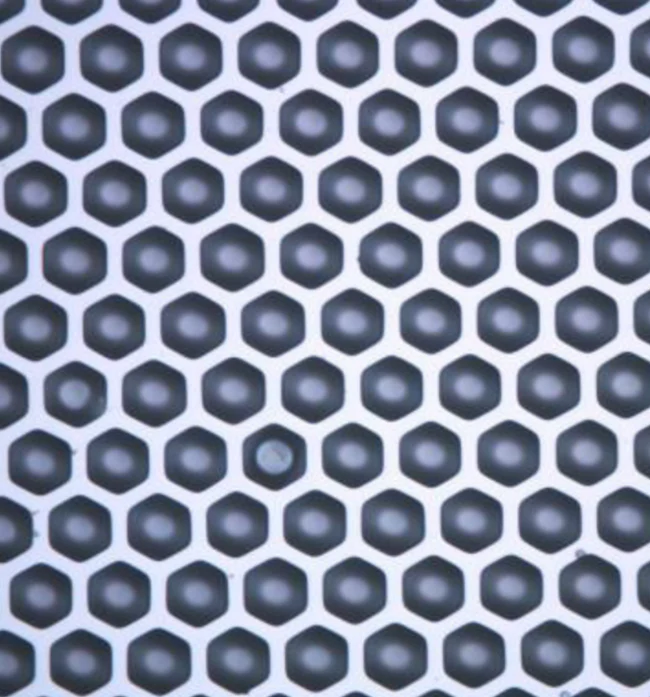
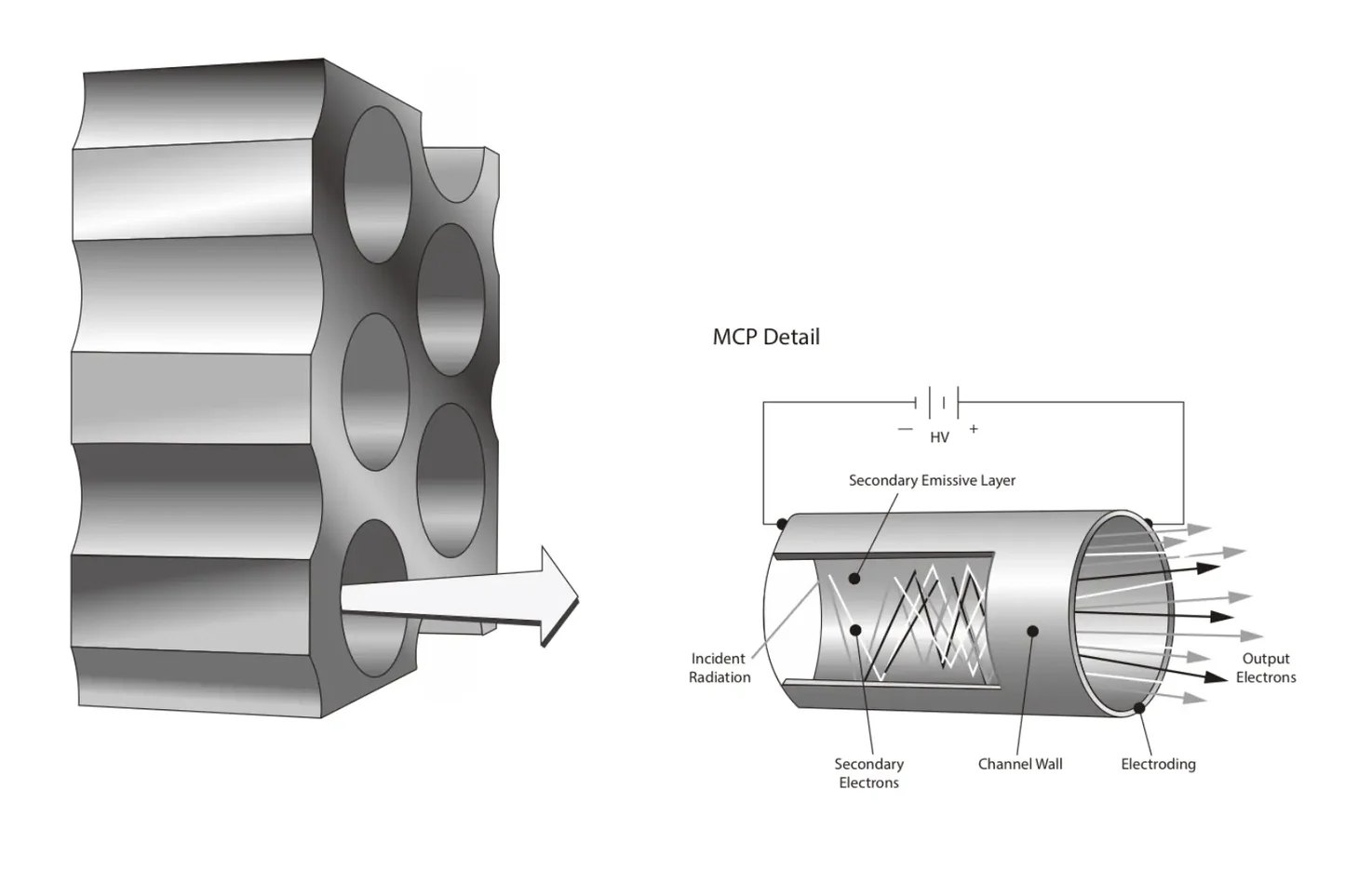
Specifications
-
Dimension of MCP
The diameter of the microchannels that determine the spatial resolution and gain of the plate.
- Typical Value: 5um,6um,8um,10um,12um
Thickness of the MCP will have impact on the Gain
- Typical Thickness of MCP is 0.3-0.5mm
-
L/D Ratio
Length of the MCP is nearly equal to the thickness of the channels.
- Typical Range: 40 - 80
The ratio of the channel length (L) to channel diameter (d) is L/D ratio.
Gain of the MCP is highly related with the L//D ratio.
-
Stacking the MCPs
The ratio of the number of output electrons to the number of input particles or photons that measures the amplification factor of the plate.
- Typical Gain for 1 Stage:
10^3 - 10^4 - Typical Gain for 2 Stages (Chevron): 10^6
- Typical Gain for 3 Stages
(Z-Stack): 10^7 - 10^8
Note: Using stacking rather than a High L/D ratio MCP.
- Typical Gain for 1 Stage:
-
Bias Angle
The angle between the normal direction and the axis of the microchannels that influences the probability of secondary emission and gain uniformity of the plate.
- Typical Value: 6° , 12°
Customizable for below angle:
- 0° - Collimation & Filtration
- 5° - Photons
- 8° - Electrons
- 12° - Ions
-
Open area ratio (OAR)
The ratio of the total area of the microchannels to the active area of the plate that indicates the fraction of incident particles or photons that can enter the channels.
- Typical Range: 55%-65%
- Max Range: 70%-80%
Note:
Higher OAR -> Primary Electrons Enter Channel Easily
-
Formats
Shapes
- Circular, Rectangular
Dimension
- Max Range: Dia100mm - Dia120mm
- Typical Range: Dia25mm - Dia75mm
- Min Range: Dia15mm
Useful Area:
- 70 - 90% of the dimension
-
Fabrication & Materials
Substrate Material
- Leadsilicate Glass
- Ni-Cr (Nickel-Chromium)
- Inconel
Coating inside the MCP
- Metallic Coating
- Dielectric Coating
-
Resistance
The resistance is the electrical resistance of the MCP material, which affects the voltage and current required to operate the MCP.
- Typical Value: 10MΩ - 300MΩ
Note:
Although lower resistance can improve theoretical performance, but it could also result in a high current and heating problem which finally degrade the performance.
-
Dark Current
The current generated by the plate in the absence of any input particles or photons that represents the noise level of the plate.
- Typical Value: 0.5 pA·cm2
-
Saturation Effects
Saturation effects in MCP are the phenomena that limit the performance and the reliability of the MCP detector when the input signal or the output voltage is too high..
-
End Spoiling
End spoiling in MCPs refers to the deposition of electrode material into the pore by up to several pore diameters at the output surface of the MCP. This technique helps focus the electrons exiting the MCP, reducing their transverse velocity. End spoiling on the output side increases spatial resolution but decreases gain.
Applications
- Image intensifiers: Image intensifiers use a MCP to amplify low-light images, such as night vision or fluorescence microscopy. The input photons are converted to electrons by a photocathode, then multiplied by the MCP, and finally converted back to photons by a phosphor screen.
- Photomultipliers: Photomultipliers use a MCP to detect and measure single photons, such as in spectroscopy or scintillation counting. The input photons are converted to electrons by a photocathode, then multiplied by the MCP, and finally collected by an anode.
- Particle detectors: Particle detectors use a MCP to detect and measure single particles, such as electrons, ions, or neutrons. The input particles are either directly multiplied by the MCP, or converted to electrons by a conversion layer before entering the MCP. The output electrons are then collected by an anode or a position-sensitive detector.
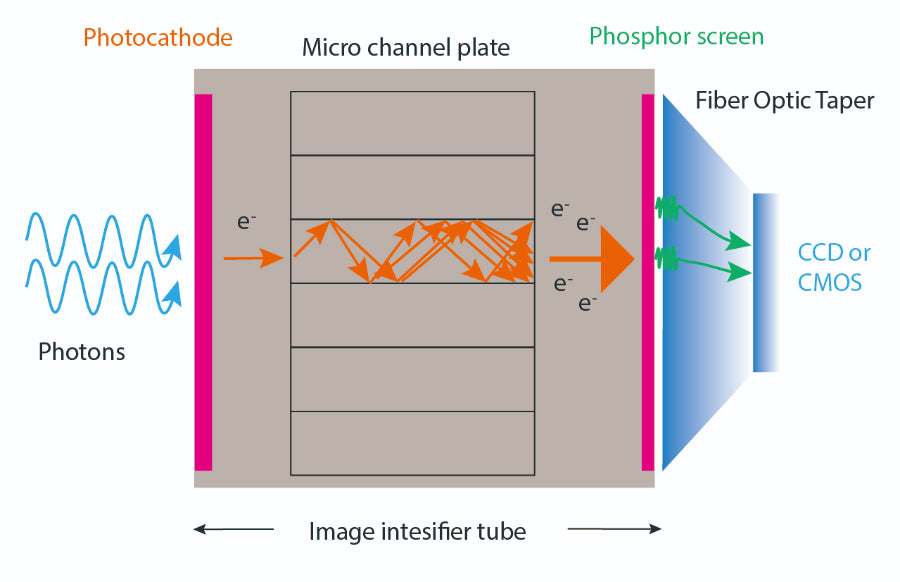
Using Microchannel plate in image intensifier
Working Principles
a fully functional MCP detector may contains the following components:
- A photocathode: This is a thin layer of material that converts the incident photons into primary electrons by the photoelectric effect. The photocathode is attached to the input window of the MCP and biased with a negative voltage.
- A microchannel plate: This is a thin wafer of glass or ceramic that contains millions of microscopic tubes (microchannels) that run parallel to each other and are perpendicular to the input and output surfaces. The inner walls of the microchannels are coated with a material that emits secondary electrons when struck by the primary electrons. The microchannels are also biased with a high voltage that accelerates the electrons towards the output end. As the electrons travel through the microchannels, they collide with the walls and produce more secondary electrons, creating a cascade of amplification.
- An anode: This is a metal layer that collects the amplified electrons from the microchannel plate and converts them into an electrical signal. The anode can have different shapes and configurations depending on the application. For example, it can be a single metal sheet, a resistive layer, a segmented array, or a phosphor screen. The anode can also provide spatial resolution by mapping the position of the incident photons on the photocathode.
- When a photon hits the photocathode, it ejects a primary electron with a certain energy and direction.
- The primary electron enters the microchannel plate and is accelerated by the electric field towards the output end.
- As the primary electron travels through the microchannel, it collides with the channel wall and emits one or more secondary electrons.
- The secondary electrons are also accelerated by the electric field and collide with the channel wall, producing more secondary electrons. This process repeats until the electron avalanche reaches the output end of the microchannel.
- The output electrons are collected by the anode and converted into an electrical pulse that corresponds to the incident photon.
Inquiry Form
Reference
-
Characterization of Polycapillary Optics Instal...
Citation Title: Characterization of Polycapillary Optics Installed in an Analytical Electron Microscope Authors: Akira Takano, Keisuke Maehata, Naoko Iyomoto, Toru Hara, Kazuhisa Mitsuda, Noriko Yamasaki, and Keiichi Tanaka Journal: JPS...
Characterization of Polycapillary Optics Instal...
Citation Title: Characterization of Polycapillary Optics Installed in an Analytical Electron Microscope Authors: Akira Takano, Keisuke Maehata, Naoko Iyomoto, Toru Hara, Kazuhisa Mitsuda, Noriko Yamasaki, and Keiichi Tanaka Journal: JPS...
-
Phase Imaging Using Focused Polycapillary Optics
Citation Opt Commun. 2016 June 15; 369: 28–37. doi:10.1016/j.optcom.2016.02.017. Keywords X-ray imaging Polycapillary optic Edge enhancement Weak attenuation model (WA) Phase-attenuation duality (PAD) Brief This article examines a technique for...
Phase Imaging Using Focused Polycapillary Optics
Citation Opt Commun. 2016 June 15; 369: 28–37. doi:10.1016/j.optcom.2016.02.017. Keywords X-ray imaging Polycapillary optic Edge enhancement Weak attenuation model (WA) Phase-attenuation duality (PAD) Brief This article examines a technique for...
-
Road to micron resolution with a color X-ray ca...
Citation Nowak, S.H., Petric, M., Buchriegler, J. et al. Road to micron resolution with a color X-ray camera – polycapillary optics characterization. Keywords X-ray fluorescence (XRF) Polycapillary optics Spatial Resolution...
Road to micron resolution with a color X-ray ca...
Citation Nowak, S.H., Petric, M., Buchriegler, J. et al. Road to micron resolution with a color X-ray camera – polycapillary optics characterization. Keywords X-ray fluorescence (XRF) Polycapillary optics Spatial Resolution...